The following is an excerpt written by Robert Schleip from chapter #9 of Erik Dalton’s book, Dynamic Body: Exploring Form Expanding Function, which features guest contributions from some of the leading thought leaders and practitioners in the massage profession. The rest of the chapter can be read in the Dynamic Body textbook, available as part of the Dynamic Lower Body home study course or as a standalone purchase.
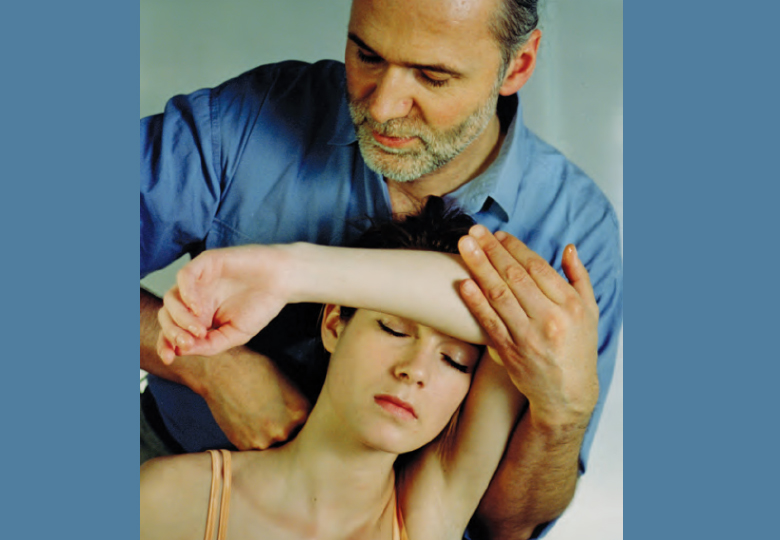
Introduction
The body-wide network of fascia is assumed to play an essential role in our posture and movement organization. It is frequently referred to as our organ of form.1 Many manual therapy approaches focus treatment on fascial tissues, and these approaches claim to alter the density, tonus, viscosity, or arrangement of fascia through the application of manual pressure.2-7 It is also assumed that these changes are not merely temporary – that they last longer than a few minutes after the immediate application. The given explanations of the involved mechanisms usually refer to the ability of fascia to adapt to physical stress. How the practitioner understands the nature of this particular responsiveness of fascia does, of course, influence the treatment. Unfortunately, fasciae are often referred to in terms of their passive mechanical properties alone. For example, experts often attribute sudden “tissue melt” to thixotropy. However, the results of recent studies seem to indicate that the application of temporary pressure – such as the pressure applied during a myofascial release session – would be unlikely to cause permanent tissue changes via such passive effects. In contrast, recent studies do seem to imply that tissue release and lasting changes may be due to an active contribution of the central nervous system and, particularly, fascial mechanoreceptors.
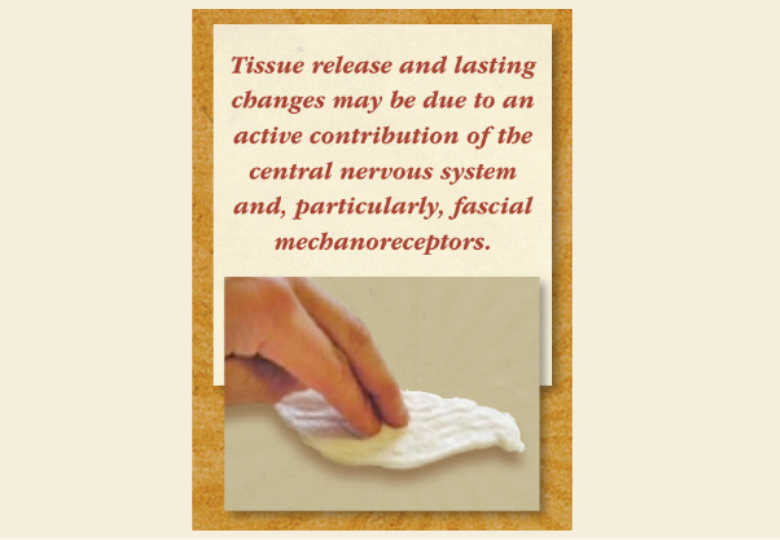
Thixotropy: The Gel-to-Sol Hypothesis
Many of the current training schools of myofascial manipulation have been profoundly influenced by Ida Rolf.6 In her hands-on work, Rolf applied considerable manual or elbow pressure to fascial structures to change their density and arrangement. Rolf pro posed the theory that connective tissue is a colloid substance in which the ground substance can be influenced by the application of energy – heat or mechanical pressure – to change its aggregate form from a more dense “gel” state to a more fluid “sol” state. Typical examples of this are common gelatin or butter, which get softer with heat or mechanical pressure. This gel-to-sol transformation – also called thixotropy8 – has, in fact, been demonstrated in connective tissues as a result of the application of long-term mechanical stress.9
However, the question arises: Is this model also useful in explaining the immediate short-term plasticity of fascia? In other words, what actually happens when a myofascial practitioner claims to feel a tissue release beneath the working hand? In most systems of myofascial manipulation, the duration of a particular “stroke” on a particular spot of tissue ranges from a few seconds to two minutes. However, practitioners often report sensing a palpable tissue release within a particular “stroke.”
Such rapid tissue transformation – less than two minutes – appears to be more difficult to explain with the thixotropy theory. As will be specified later, stud ies on the subject of “time and force dependency” of connective tissue plasticity suggest that either much longer amounts of time or significantly more force are required for permanent deformation of dense connective tissues.10 Furthermore, the problem of reversibility arises. In colloidal substances, the thixotropic softening effect lasts only as long as the pressure or heat is applied. Within minutes after the heat or force application, the substance returns to its state of previous rigidity – think of the butter in the kitchen. The temporary nature of the achieved tissue softening is, therefore, not an attractive implication of this theory for the practitioner.
Piezoelectricity: Fascia as a Liquid Crystal
James Oschman and others have added piezoelectricity as an intriguing explanation for fascial plasticity.11,12 When an outside mechanical pressure temporarily separates the electric centers of neutrality on the inside of a crystal lattice, a small electric charge can be detected on the crystal’s surface. This effect is called piezoelectricity. Since connective tissue can be seen to behave like a “liquid crystal”13 these authors propose that the cells that produce and digest collagen fibers (fibroblasts) might be responsive to such electric charges. To put it simply: Pressure from the outside creates a higher electric charge, which stimulates the fibroblasts to alter their metabolic activity in that area.
However, the processes involved seem to require time as an important factor. The half-life span of non-traumatized collagen has been reported to be 300 to 500 days, and that of ground substance 1.7 to seven days.3 While it is definitely conceivable that the production of both materials could be influenced by piezoelectricity, both life cycles appear too slow to account for immediate tissue changes that are significant enough to be palpated by the working practitioner.
Fascial Plasticity: Traditional Explanations Insufficient
Both models, thixotropy and piezoelectricity, are appealing explanations for long-term tissue changes. Nevertheless, it seems they are not sufficient to account for the short-term plasticity of fascial tissues. Laboratory studies on the subject of time- and force dependency of connective tissue plasticity (in vitro, as well as in vivo) have shown the results outlined below. In order to achieve a plastic elongation of dense connective tissues, one needs to apply: a. either an extremely forceful stretch of three to eight percent fiber elongation, which will result in tissue tearing, inflammation, and other side effects that are usually seen as undesirable in a myofascial session. As an example, for an 18-millimeter-wide distaliliotibial band, such permanent elongation starts at 60 kilograms (kg).14 b. or it takes more than an hour (which can be taken at several intervals) with a less brutal one to 1.5 percent fiber elongation, if one wants to achieve permanent deformation without tearing and inflammation.10,14
Image 1. illustrates the typical relationships for short-term strain applications. Microfailure is seen as the breaking of some individual collagen fibers and some fiber bundles, resulting in a permanent (plastic) elongation of the tissue structure. This is usually followed by a cycle of tissue inflammation and repair. Based on measurements with different kinds of paraspinal tissues, A. Joseph Threlkeld, a professor of physical therapy, calculates that microfailure of most dense connective tissues occurs at around 224 to 1,136 N, which equals 24 to 115 kg.14 While high velocity thrust techniques might create forces within that range, it seems clear that the slower soft tissue manipulation techniques are hardly strong enough to create the described tissue response.
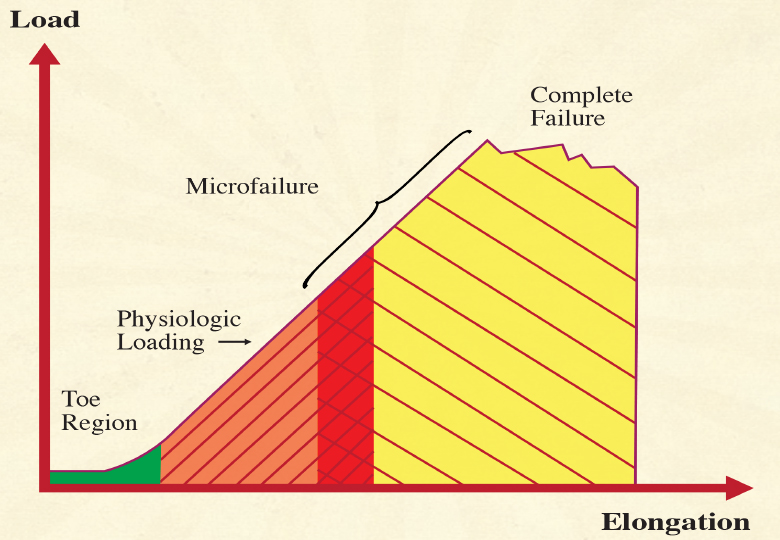
Look at the stress-strain curve of dense connective tissue illustrated above (Image 1.) Most forces generated during daily life load the tissue in the linear region of the curve and do not produce any permanent elongation. Microfailure with permanent elongation happens at extreme loads only and is accompanied by tearing and inflammation. The region of overlap of the microfailure zone with the physiologic loading zone varies with the density and composition of the tissue, yet for most dense connective tissues, it would be well above a 20 kilogram loading.15
In other words, while it may be possible to induce lasting changes in very soft connective tissues, such as in the areolar layer of the subcutis, the force/time dimensions used in myofascial release sessions are hardly sufficient to induce sustaining deformations in dense fascial sheets, which are usually the main focus of myofascial release sessions.
A simple thought experiment may be used for corroboration. During everyday life, the body is often exposed to loading magnitudes similar to the manual pressures in a myofascial treatment session. While body structure may adapt to long-term furniture use, it is almost impossible to conceive that adaptations could occur so rapidly that any uneven load distribution in sitting – for example, while reading this book – would permanently alter the shape of your pelvis within a minute. It seems essential, therefore, that we find additional explanations – besides the thixotropic and piezo electric ones – to account for the palpable tissue changes that occur in a treatment session.
The Nervous System as a Rapid Self-Regulatory System
From an evolutionary perspective, it makes sense that animals have a slowly adapting plasticity system in order to adjust to patterns of long-term use. In addition to this capacity, they have also developed a more rapid system of adapting their form and local tissue density to temporary demands. Such a rapidly adapt ing system is likely to play a key role in the lasting tissue changes reported by manual therapists. The question then becomes: Which systems allow for this rapid adaptation?
From observation, we know that an animal’s rapid regulation system is capable of adapting to how the animal perceives its interaction with the environment. The author’s own experiments in treating anesthetized people – which can give very similar results as manually treating fresh pieces of raw meat – have shown that myofascial release work does not affect tissues in the same way as it would if the person possessed intact neural regulation.16
Therefore, it seems plausible that this ability to quickly adapt to low-force manual manipulation is mediated by a finely tuned coordination system that is involved in the perception of our environment, as well as in sensing our own internal needs at any time. Traditionally, this body system has been called the nervous system.
The inclusion of the nervous system in attempting to understand fascial responsiveness is hardly a new concept altogether. Andrew Taylor Still, the founder of osteopathy, wrote more than a century ago: The soul of man with all the streams of pure liv ing water seems to dwell in the fascia of his body. When you deal with the fascia, you deal and do business with the branch offices of the brain, and under the general corporation law, the same as the brain itself, and why not treat it with the same degree of respect.17
The inclusion of the nervous system in attempting to understand fascial responsiveness is hardly a new concept altogether.
Even so, many people discount the nervous system in the discussion of fascial responsiveness, because they consider the human nervous system to be organized like an old-fashioned telephone switchboard from the industrial age. Such a system would then, of course, be incapable of representing finer and more complex processes such as “life energy,” intuitive insights, rapid movement refinements, or human empathy.
The reader is cordially invited to consider this to b an outdated model. Current concepts in neurobiology primarily view the brain as a liquid system, in which fluid dynamics of a multitude of liquid and even gaseous neurotransmitters have come to the forefront.
It is a common opinion in the field that fascia must contain a different and much faster communication system than the nervous system. Those who hold this view often believe that the nervous system cannot adapt quickly enough for the rapid responses in human behavior. Thus, the fascia must have a separate, faster adaptation system. However, transmission of impulses in our nervous system often happens via messenger substances that travel along neural pathways, as well as through the blood, lymph, cerebro-spinal fluid, or ground substance.18
This global system for rapid body regulation is in separably connected to the endocrinal and immune systems, and it also works with complex feedforward system dynamics. Rather than picturing the nervous system as a hard-wired electric cable system – which in the view of many bodyworkers is then, of course, incapable of being involved in more subtle energetic phenomena – picture it in your mind’s eye as a wet tropical jungle.19
This jungle is a self-regulatory field – involving complex feedforward system dynamics – with an amazing amount of complexity, plasticity, and continuous reorganization. Such a complex neural field could easily be involved in the rapid fascial changes experienced during manual therapy.
The Nervous and Fascial Systems are Inseparably Interwoven
Our central nervous system receives its greatest amount of sensory input from myofascial tissues. Unfortunately, the neural dynamics of fascia have rarely been explored in detail. The majority of these sensory neurons within myofascial tissues are so small and complex that, until recently, little has been known about them.20
If we discover exactly how the nervous system connects with fascia, we will be in a better position to uncover the causes of patients’ myofascial release during passive bodywork sessions. Fascia contains four types of sensory nerve endings, which are responsive to mechanical stimulation:
- Golgi organs
- Ruffini receptors
- Pacini corpuscles
- Interstitial receptors
Together, these sensory nerve endings can be called fascial mechanoreceptors, which means that they respond to mechanical tension and/or pressure. Such mechanoreceptors have been found in intramuscular, as well as extramuscular, fascial tissues. Research has shown that stimulation of fascial mechanoreceptors triggers changes in the nervous system, as well as global muscle relaxation. For example, it has been demonstrated that deep mechanical pressure to the human abdominal region21 or sustained pressure to the pelvis22 produce parasympathetic reflex responses,a including synchronous cortical EEG patterns, increased vagal activity (which causes a decrease in heart rate), and decreased EMG activity.
According to the model of hypothalamic tuning states, developed by Ernst Gellhorn, this in crease in vagal tone not only triggers autonomic nervous system changes and related inner organs, but it also tends to activate the anterior lobe of the hypothalamus. Such a “trophotropic tuning” of the hypothalamus then induces more quiet emotional activity, an increase in synchronous cortical activity, and a lower overall muscle tonus, or softening of the muscles.23
In other words, deep mechanical pressure to these areas causes a person to be more relaxed. Therefore, it appears that deep manual pressure – specifically if it is slow or steady – stimulates particular fascial mechanoreceptors, which results in an increase of vagal activity.
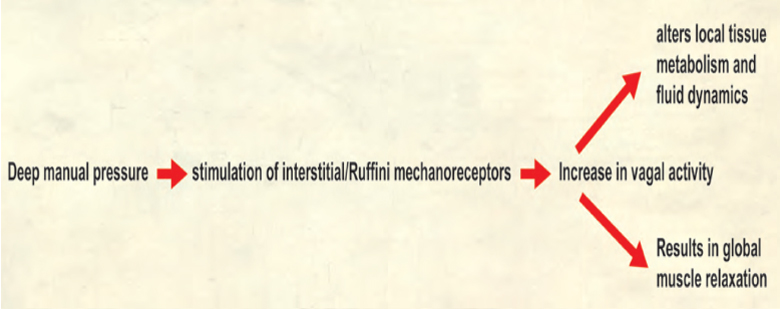
The most likely candidates for such receptors are the Ruffini endings, as well as interstitial receptors in fascial tissues. This increase in vagal activity then not only induces an alteration in local fluid dynamics and tissue metabolism, but it also results in global muscle relaxation, as well as a more peaceful mind and less emotional arousal.
In addition, mechanoreceptors are also found abundantly in visceral ligaments, as well as in the duramater of the spinal cord and cranium. It, therefore, seems quite plausible that many of the benefits of osteopathy on these areas could be sufficiently explained by a stimulation of mechanoreceptors. Such effects can explain profound autonomic changes without the need to rely on more esoteric assumptions, such as a breathing cerebrospinal liquid system.24
About the author
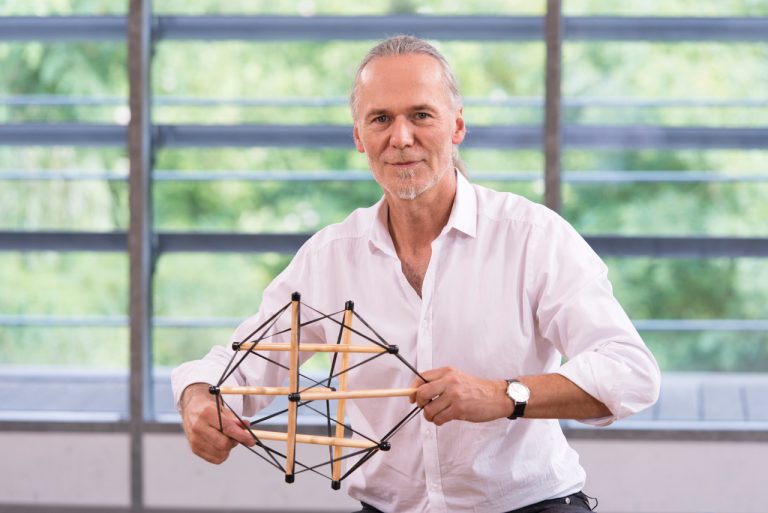
Robert Schleip is the director of the Fascia ResearchProject, part of the division of neurophysiology at Ulm University in Germany. In 2007, he received the Vladimir Janda Award for Musculoskeletal Medicine for his pioneering work on active fascial contractility. Schleip holds a doctorate in human biology and a master’s in psychology. He has been a Certified Rolfer ® since 1978 and currently serves as research director for the European Rolfing Association. Schleip is also a Rolfing instructor and Feldenkrais® teacher, and he has been teaching anatomy for more than 25 years. www.fasciaresearch.de
References
1. Varela, F.J., & Frenk, S. (1987). The organ of form: towards a theory of biological shape. J Social Biol Struct, 10, 73-83.
2. Barnes J.F. (1990). Myofascial Release: The Search for Excellence. Paoli, PA: Rehabilitation Services Inc.
3. Cantu, R.I., & Grodin, A.J. (1992). Myofascial Manipu lation: Theory and Clinical Application. Gaithersburg, MD: Aspen Publishers.
4. Chaitow, L. (1980). Soft-Tissue Manipulation. Roches ter, VT: Healing Arts Press.
5. Paoletti, S. (1998). Les fascias – Role des tissues dans la mecanique humaine. Vannes cedex, France: Le Prisme.
6. Rolf, I.P. (1977). Rolfing: The Integration of Human Structures. Santa Monica, CA: Dennis-Landman.
7. Ward, R.C. (1993). Myofascial Release Concepts. In J.V. Basmajian and R.E. Nyberg (Eds.), Rational Manual Therapies. Baltimore, MD: Williams & Wilkins.
8. Juhan, D. (1987). Job’s Body: A Handbook for Body work. Barrytown, NY: Station Hill Press.
9. Twomey, L., & Taylor, J. (1982). Flexion, creep, dys function and hysteresis in the lumbar vertebral column. Spine, 7(2), 116-122.
10. Currier, D.P., & Nelson, R.M. (1992). Dynamics of Hu man Biologic Tissues. Philadelphia, PA: F.A. Davis Com pany.
11. Oschman, J.L. (2000). Energy Medicine. Edinburgh, United Kingdom: Churchill Livingstone.
12. Athenstaedt, H. (1974). Pyroelectric and piezoelectric properties of vertebrates. Ann NY Acad Sci, 238, 68-110. 13. Juhan, D. (1998). Job’s Body: A Handbook for Bodywork. Barrytown, NY: Station Hill Press.
14. Threlkeld, A.J. (1992). The Effects of Manual Therapy on Connective Tissue. Phys Ther, 72(12), 893-901.
15. Chaudhry, H., Schleip, R., Zhiming, J., Bukiet, B., Maney, M., & Findley, T. (2008). Three-Dimensional Mathematical Model for Deformation of Human Fasciae in Manual Therapy. J Am Osteopath Assoc, 108(8), 379-390.
16. Schleip, R. (1989). A new explanation of the effect of Rolfing. Rolf Lines, 15(1), 18-20.
17. Still, A.T. (1899). Philosophy of Osteopathy. Kirks ville, MO: Academy of Osteopathy.
18. Kandel, E.R. (1995). Essentials of neural science and behavior. New York, NY: Appleton & Lange.
19. Schleip, R. (2000). Lichtblicke im Dschungel der Gehirnforschung. FeldenkraisZEIT, 1, 47-56.
20. Engeln, H. (1994). Konzert der Muskeln und Sinne. GEO Wissen, 1(Mai), 90-97.
21. Folkow, B., Gelin, L.E., Lindell, S.E., Stenberg, K., & Thoren, O. (1962). Cardiovascular Reactions Dur ing Abdominal Surgery. Ann Surg, 156(6), 905-913.
22. Koizumi, K., & Brooks, C.M. (1972). The integra tion of autonomic system reactions: a discussion of autonomic reflexes, their control and their associa tion with somatic reactions. Ergeb Physiol, 67, 1-68.
23. Gellhorn, E. (1967). Principles of Autonomic Somatic Integrations: Physiological Basis and Psychogical and Clinical Implications. Minneapolis, MN: University of Minnesota Press.
24. Arbuckle, B.E. (1994). Selected Writings. Indianapolis, IN: American Academy of Osteopathy.
On sale this week only!
Save 25% off the "Dalton Technique Treasures" eCourse
The “Dalton Technique Treasures” eLearning course is a compilation of some of Erik’s favorite Myoskeletal Alignment Techniques (MAT). Learn MAT techniques to assess and address specific sports injuries, structural misalignment, nervous system overload, and overuse conditions. ON SALE UNTIL July 29th! Get Lifetime Access: As in all our eLearning courses, you get easy access to the course online and there is no expiry date.